Commissioning of the spectroscopic mode of NOTcam
NOTcam was designed such that grisms and slits can be mounted and
rotated into the beam to quickly switch from imaging mode to
spectroscopy and vice-versa. We have done extensive tests in order to
prepare for the spectroscopic mode. The tests were followed by first-light observations in August 2003, for
the low-res spectroscopic mode of NOTcam. Since then, this mode has
been offered to the community.
In May 2005, we obtained first-light
observations for the medium-resolution spectroscopic mode of NOTcam.
This mode can now also be applied for.
Nevertheless, further tests still remain to be done as part of the
ongoing commissioning of the spectroscopic mode of NOTcam. Action
points are indicated by a small dot, below.
Slits
Slits and other aperture-wheel items were purchased a several years
ago. The slits are made of very thin nickel-copper laminate, with the
slits cut in the nickel part. The slits were originally mounted in
aluminium holders. When mounted in NOTcam the slits can be physically
examined as they sit very close to the entrance window. It turned out
that when cold, the slits are deformed due to the differential
expansion of the copper and nickel, and the aluminium holders.
In 2004 new copper slit holders were installed for the 128 and 64
micron slits, which made the deformations less apparent.
One of the 4 slits is clearly wrinkled because of the above effect,
but proves to be useful as the jaws remain quite parallel. Although
the slit width is 128 micron according to specifications, it is imaged
to about 2-2.5 pixels FWHM (instead of 4 pixels) on the detector by the
Wide-Field Camera of NOTcam. This slit is usable for point
sources. The 64 micron slit, designed for
2-pixel resolution, is actually imaged onto much less than 2 pixels,
and should not be used.
This plot shows flatfielded H-band
lamp-light images of these two slits summed over the width
of the slit, to indicate the deformation from parallel of the slit
jaws. The slit transfer functions are normalized such that the Y axis of
the plot indicates the effective slit width.
Also for the HRC mode we have only one useful slit, and also here the
slit that was originally designed to be imaged onto 4 pixels, is
actually giving about 2.5-pixel resolution. This
plot shows the slit transfer function of the 44 micron slit,
again normalised such that the Y axis of the plot indicates the
effective slit width. The slit is suitable to be used for observations.
The grism
So far one grism has been designed by Michael Andersen, and this grism
is installed in NOTCAM. The grism has an echelle grating and is designed to give intermediate resolution
(2-pixel R=2500, with dispersion 2.5-4.1 Angstrom/pixel) in J (5th and
6th order), H (4th order) and K (3rd order) when used with the WFC.
In combination with the HR Camera the resolution will be about 3 times
higher, but the sampled wavelength range will be about 3 times less.
The grism is used in combination with the JHK filter set in NOTcam.
The grism was delivered in July 2003, and was mounted in a holder made
by Niels Michaelsen in Copenhagen. This plot shows the throughput of the grism as measured by the
manufacturer (note that the order overlap has not been accounted
for).
Proposed upgrade: we could purchase low-resolution
grisms spanning ZJ and HK bands.
An HK filter is already purchased for NOTcam (filter #226),
but a ZJ filter needs
to be ordered together with the new grisms.
Internal focus
In order to determine the optimal focus, and the optimal distance
between the aperture wheel items and the collimator, we made a set of
Hartmann masks which were mounted in the stop wheel. Using the masks
it turned out that there is a considerable chromatic focus variation.
This plot shows the internal focus of NOTcam as
a function of wavelength for a slit mounted 2mm towards the collimator
in combination with the WFC (before the aperture wheel assembly was
moved).
With the grism in the beam the internal focus has to be upped by 200
units for the WF Camera (with respect to no grism).
In the end of 2004, the aperture wheel assembly was machined to allow
it to be moved 2mm away from the collimator (towards the sky). This
was needed to be able to focus aperture wheel items (e.g. slits, pinholes)
with the HRC. The tables below reflect the internal focus of WFC and
HRC slits, after the movement of the aperture wheel assembly.
HRC + 44-micron slit (no grism) | band |
internal focus | FWHM | telescope focus |
Z | >6000 outside range | 4.5 pixel |
|
J | 5450 | 2.6 pixel |
|
H | 3000 | 2.6 pixel |
|
K | 1000 | 3.1 pixel |
|
WFC + 64-micron slit (no grism) | band |
internal focus | FWHM | telescope focus |
Z | 4950 | |
|
J | 4600 | |
|
H | 4300 | 1.5 pixel |
|
K | 4050 | |
|
WFC + 128-micron slit (no grism) |
band | internal focus | FWHM | telescope focus |
Z | 5150 | | ~23200 +/- 100 |
J | 4750 | | ~23200 +/- 100 |
H | 4450 | 2.6 pixel | ~23200 +/- 100 |
K | 4250 | 3.4 pixel | ~23200 +/- 100 |
Notes to table:
all Z-band values were obtained using filter #222 ( Yn )
for the 1mm pinhole + WFC use the same focal settings as WFC + 128-micron slit
for WFC the 50-micron pinhole cannot be focussed: in J the focus should be ~6700
It turned out that in order to be able to properly focus the
High-Resolution Camera, the aperture wheel assembly had to be moved
away from the collimator, and the slits have to be mounted in a
special holder such that they are as far away as possible from the
collimator. The only way to achieve the mounting of this holder was
to use one of the 4 big aperture wheel hole positions.
The slits for use with the WFC, however, needed to be positioned
further towards the collimator by about five millimeters (with aperture
wheel moved) in order to get proper focus over the full ZJHK wavelength
domain. The first-light observations were carried out in good seeing
conditions, and indicated that for the WFC spectra there is no clear
evidence for astigmatism caused by the positioning of the slits with
respect to the collimator.
For the HRC the on-sky measurements also show no evidence that
the displacement of the slit adversely affects the imaging quality.
Instrument flexure
Using the small pinhole and the WFC, flexure tests showed that slit-shifts of up to 4 pixels can be expected when
slewing to different sources at high airmass for random rotator
angles. While keeping the rotator angle fixed, e.g. following one
source using the parallactic angle, the slit
shifts are usually less than 1 pixel.
For the parallactic angle the shifts are mostly along the slit.
These flexure slit-shifts are generally much larger than the shifts
introduced by the (re)positioning of the aperture wheel. We have
tested the repeatability of the slit position after moving the
aperture wheel. We used the HR Camera with the telescope at
zenith. Here, the slit ran parallel to the columns of the detector (Y
direction), with the rotation of the aperture wheel introducing shifts
in the X direction. Scatter between subsequent X-positions of the
slit is about 0.1-0.2 HR pixels (0.01-0.02 arcsec), however sometimes
a larger step of about 0.5 HR pixels occurs (0.04 arcsec). The large
step may correspond to the motor of the aperture wheel losing track of
1 stepper motor unit.
Considering the above there is no clear preferred direction for the
slit, i.e. along detector columns or rows, as flexure-induced shifts
are much more important than shifts introduced by the (re)positioning
of the aperture wheel. It is clear that wavelength calibration frames
will have to be obtained at each target position on the sky.
Considering the detector cosmetics, we have decided that the preferred
slit position is horizontal, such that the spectra run along the
columns of the array.
Note that for a horizontal slit the parallactic angle has TCS
rot-pos = -90 or 90. For vertical slits: rot-pos = 0 or 180.
Wavelength calibration
NOTcam originally had no dedicated calibration unit. We investigated the
possibility of using the OH sky lines for on-line wavelength
calibration. For the H and K bands exposure times on the order of 200
seconds (for WFC) give sky-lines with enough flux that they are suitable for
wavelength calibration. For very bright stars the on-target spectra
(with short exposure times) will not be suitable to do wavelength
calibration. These plots give identifications of the OH lines in
vacuum for the H-band and for the Ks band. The scatter of the position of the line
centroids around the wavelength solution (3rd degree polynomial) is
less than 1 Angstrom.
For the J-band exposure times on the order of 200 seconds do not give
suitable sky-lines with enough flux. For this purpose we have
experimented with calibration lamps normally used with ALFOSC. As
part of the experiment the ZnAr and Ne lamps were hung above NOTcam
at a distance similar to that of the flatfield lamp inside the
telescope baffle. Although these particular lamps do not emit useful
lines in H and K, they did provide lines with which the J band can be
calibrated.
As of 2005, we have a calibration unit in the telescope baffle, that
can be used for NOTCAM spectroscopy. In order to use the lamps, the
mirror-covers need to be closed, introducing extra overheads. The unit
contains a flatfield lamp that is also used for ALFOSC, and a Xenon
and Argon lamp. Currently the lamps can be switched on in the control
room manually. Arc maps can be found here.
Proposed upgrade: a new calibration unit that swings in the beam
fast, and that is completely operated under computer control, is
desired.
Wavelength stability
For the first-light data in the H-band the wavelength solution was not
stable: the dispersion changing by 0.25% between exposures taken 1
hour apart, but at similar sky position and rotator angle. Could be a
small tilt of the grism on the order of 0.2 degree. Or else flexure
within the camera. Or movement of the slit from/to collimator? Needs
testing!
During further tests it was noted that when moving the telescope the
wavelength zeropoint and the dispersion can change on the 1% level,
which effectively means that both wavelength calibration arc frames
and fringe correction flats have to be obtained at each and every
telescope pointing.
Further test the stability of the dispersion.
Investigate whether the grism can be mounted better.
System efficiency
Some preliminary results from spectroscpic standards can be found here.
Flatfielding and fringes
A flat field lamp is situated inside the telescope baffle. The lamp
flats are a necessary means to get rid of the quite substantial
pixel-to-pixel variations of the detector. From the first-light
observations we found that one can achieve a signal to noise ratio of
S/N=80 in the spectral continuum using standard reduction steps. The
problem with the baffle lamp is that the mirror covers have to be
closed in order for correct operation.
Using the flatfield lamp inside the telescope baffle a clear ripple pattern becomes immediately present.
These ripples are probably due to interference in the 330 micron thick
sapphire detector substrate. The fringe wavelength is linear with
wavelength of the incident light.
band | fringe wavelengths | peak-to-peak amplitude (WFC+128-micron slit) |
old engineering array | new science array |
J | 5.5 pixel | 17% | 9% |
H | 6.7 pixel | 18% | 10% |
K | 8.8 pixel | 19% | 13% |
Fringe amplitudes are highest in the central parts of the array.
The fringes are around 25% peak-to-peak when the HRC with the 44-micron
slit is used (for the old engineering array !).
Bad pixels, hot pixels, and hot rows
Bad pixels, hot pixels, and hot rows are discussed in this report.
On dark frames, and frames with low count levels, such as the sky part
of spectroscopic images or on wavelength calibration images, hot rows
are clearly visible (see this
report or the first-light
report). The count level in the hot rows does note scale with
exposure time. In fact, the hot rows behave more like rows with a
deviant zero level.
The number of hot pixels increases with exposure time (see
table below).
For exposures of more than 5 to 10
minutes the hot pixels are so abundant that they may inhibit proper
reduction of the spectra. The number of hot pixels for long exposures
is similar when using either reset-read-read or ramp-sampling mode.
Percentage of hot pixels (i.e. more than 8 sigma above
background) in the central 250x250 pixels of the top-left quadrant
(Q3).
Exptime [s] | 0 |
3 | 9 |
27 | 81 |
243 | 729 |
Hot pixels [%] | 0.1 |
0.2 | 0.3 |
0.6 | 1.0 |
1.8 | 2.5 |
An unfortunate feature of the new science array is the presence of
clusters of hot pixels. In long dark exposures (see the 729 sec dark
expo below) these patches of hot pixels appear as stellar images:
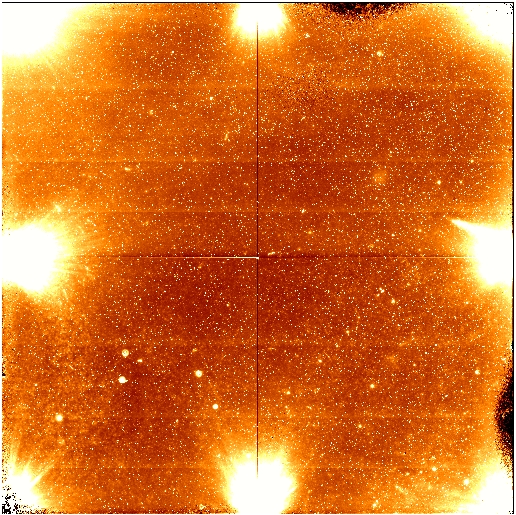
Some of the clusters reach saturation and should always be avoided.
Columns to be avoided are (in MEF format):
x=110-120, 235-250, 390-400, 415-425, 715-765, 870-880, 945-955
Calibration data for spectral reduction
A simple ABBA nodding of the telescope is effective in removing the
background. Subtracting the B exposures from the A exposures does
leave a residual background level, while removing most of the
small-scale detector background features. The residual background
level can be fitted away if the nodding step is large enough. For very
bright stars the wings of the spatial profile can be traced to +/- 30
pixels with the WFC. This means that the ABBA nodding step should be
20-25 arcsec for correct background subtraction. For fainter stars
the nodding step could made be smaller.
In order to get optimal extraction to work, the 2D images first have
to be corrected using a bad-pixel map. Interpolation across bad pixels
provides the first rough correction that the optimal extraction
algorithm cannot handle. A bad-pixel map can easily be constructed
from two flat fields with different exposure levels. This map should
be (re-)made for each observing run. Even for very bright stars
variance weighting and cleaning of deviant pixels is worth doing.
The lamp flats are essential to get rid of the substantial
pixel-to-pixel variations of the detector, and should be obtained
after each telescope pointing.
Wavelength calibration data should be obtained after each telescope
pointing.
For the first-light WFC observations it was possible to correct for
telluric lines and at the same time for the fringes by using a nearby
featureless comparison star. The S/N in the continuum was around 80
after this correction.
For horizontal slits, the sky lines run along the rows of the
detector. For sky-line based wavelength calibration one needs to
extract these sky lines. The sky lines are bent because of optical
distortion, and run into bands of hot rows. It should be possible to
subtract out the hot rows using dark frames. Note that the counts in
the hot rows do not scale with exposure time of the dark frames.
The dark frames show different and non-linear background levels for
different exposure times. It is a good idea to obtain a set of dark
frames with the same exposure times as used for the science and other
calibration frames.
We need to obtain more flux standard observations for
throughput/efficiency determination.
John Telting
|